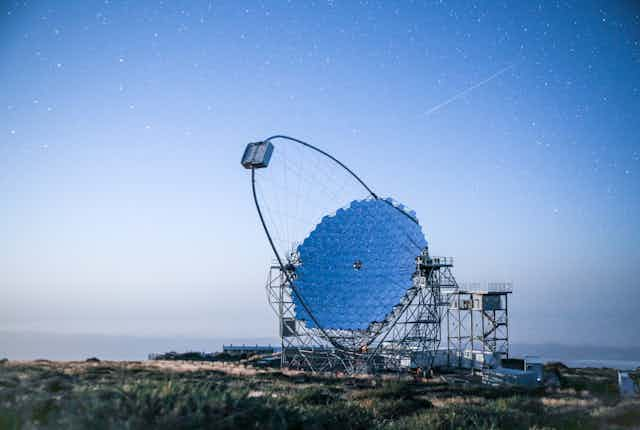
Long gone are the days when astronomers only studied the skies with simple optical telescopes. Today, unveiling the mysteries of the Universe involves ever-larger and more complex facilities that detect things like gravitational waves and different forms of electromagnetic radiation – the spectrum of energy that includes visible light and X-rays.
One particularly specialised branch of astronomy is gamma-ray astronomy. It does what is says on the tin, searching for gamma rays, which are the most energetic photons (light particles) on the electromagnetic spectrum. In fact, they are millions of times more energetic than the light we can see.
In astronomy, gamma rays are produced by some of the hottest, most energetic events in the universe, such as star explosions and black holes violently “feeding” on surrounding matter. While gamma rays are now linked to dozens of different types of sources, in many cases we still don’t know conclusively what kinds of energetic particles are creating these rays.
Excitingly, gamma-ray astronomy is due to get a massive leg up with a new facility. Once the globally distributed Cherenkov Telescope Array (CTA) is complete, it will view the gamma-ray sky with ten times more sensitivity than what’s currently possible.
With more than 60 telescopes, the CTA is expected to provide deep insight into the nature of dark matter – an invisible, hypothetical type of matter making up about 85% of the mass of the Universe. The array could also help solve one of the longest-running mysteries in astronomy: where cosmic ray particles (energetic nuclei and electrons in our galaxy and beyond) come from. Gamma rays are linked to these particles, providing a means to trace them.
Read more:
Why do astronomers believe in dark matter?
Flashes from outer space
Gamma-ray astronomy was born in the early 1960s as space-based satellites were developed to look for energetic radiation from outer space.
NASA’s Fermi mission, launched in 2008 to a low-Earth orbit, has so far catalogued several thousand gamma-ray sources. The Fermi spacecraft continues to provide 24-hour live coverage of the sky, measuring gamma rays with energies reaching several 1,000 giga-electron volts in energy. That’s about one trillion times the energy of visible light.
To study gamma rays with even higher energies, we need to use ground-based methods. Although Earth’s atmosphere shields us against radiation from outer space, we can still detect the secondary effects of this shielding on the ground.
That’s because when a gamma ray interacts with Earth’s atmosphere, it sparks an electromagnetic cascade or “air shower” of more than a billion secondary particles. These particles are mostly electrons and their anti-matter partners, called positrons. These air showers contribute about 30-50% of the natural radiation we experience in our lives.
CTAO/ESO, CC BY
Making the invisible visible
While nothing can go faster than the speed of light in a vacuum, charged particles such as electrons and positrons (anti-electrons) can actually move faster than light when moving through air.
When this happens, a shockwave is created as a flash of blue and ultraviolet light. This flash, called Cherenkov radiation, is named after Soviet physicist Pavel Cherenkov who first detected the phenomenon in 1934 (and received the 1958 Nobel Prize in Physics for it alongside two colleagues). The blue glow of Cherenkov radiation can be seen in water cooling ponds surrounding nuclear power reactors.
Parilov/Shutterstock
At ground level, telescopes with large mirrors and sensitive cameras can detect the Cherenkov light produced by a gamma ray striking our atmosphere. These cameras need just about ten nanoseconds to capture a Cherenkov flash against the bright background of starlight and moonlight.
The first Cherenkov telescopes were developed in the 1960s. After many variants, it was the Whipple Telescope in the United States that in 1989 discovered gamma-ray photons coming from the Crab Nebula.
This was the first time gamma rays with energies of more than 1,000 giga-electron volts (or 1 tera-electron-volt, TeV) were detected. Thus, tera-electron-volt gamma-ray astronomy was born.
Searching for the extremes
Today, all three of the world’s best TeV gamma-ray facilities – HESS in Namibia, MAGIC in La Palma, Spain and VERITAS in Arizona – have discovered more than 200 TeV gamma-ray sources. These powerful rays are linked to cosmic regions of particle acceleration, such as pulsars, supernova remnants, massive star clusters, and supermassive black holes in the Milky Way and other galaxies.
HESS has shown our Milky Way galaxy is rich in TeV gamma-ray “light”, including in the centre of the galaxy.
TeV gamma-rays are also seen from mysterious gamma-ray bursts and other fleeting, transient events. These are now informing our understanding of the extreme conditions in which gamma rays are created.
The next-generation CTA will use the lessons learnt from HESS, VERITAS and MAGIC, by extending the number of telescopes deployed on the ground to over 60 telescopes. CTA will also use a combination of three different telescope sizes optimised for three gamma-ray energy bands, providing unprecedented performance and “sharpness”.
It will have arrays at two sites on the ground: one in Paranal, Chile (51 telescopes) in the Southern Hemisphere, and one in La Palma (13 telescopes) in the Northern Hemisphere.
CTA has attracted membership from more than 1,000 scientists, including Australian scientists from seven universities. It’s progressing well, with the first northern telescope already detecting gamma rays from the Crab Nebula and several gamma-ray flares from active galaxies powered by supermassive black holes.
Within a few years we expect to see the first southern telescopes also detecting gamma rays, yielding many more discoveries. With CTA, we will have new insights into where extreme particle acceleration is taking place in our Milky Way.
Read more:
New era of astronomy uncovers clues about the cosmos
Post a Comment